- About
- Organization
- Organization Overview
- Dean’s Office
- Department of Bioengineering and Therapeutic Sciences
- Department of Clinical Pharmacy
- Department of Pharmaceutical Chemistry
- Quantitative Biosciences Institute
- Org Chart
- Research
- Education
- Patient Care
- People
- News
- Events
Yang study demonstrates simulator to study antibiotic dosing against biofilms
By David Jacobson / Thu Oct 29, 2015
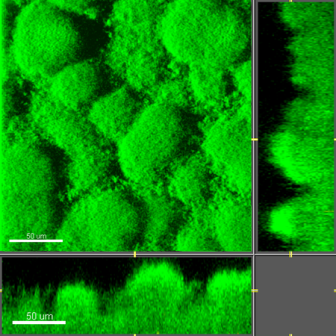
Katherine Yang
In these multiple images of P. aeruginosa biofilm a bacterial community that takes the form of mushroom-like structures. The main, upper left image looks down at the biofilm, the lower, right-hand images show cross-sections. This biofilm was cultured from the airways of a patient with cystic fibrosis.
Nearly every human bacterial infection—including some of the most serious, life threatening, and costly to treat—can take the form of a biofilm, in which bacteria aggregate into structured communities that enclose themselves within a secreted slime.
Biofilms can be up to 1,000 times more resistant to antibiotics than free-swimming (planktonic) bacteria. But antibiotic regimens are typically dosed based on antibiotic testing done against planktonic bacteria in liquid cultures. Thus optimal dosing to eradicate biofilm infections, from those on orthopedic implants to those in the lungs of cystic fibrosis patients, is largely unknown.
Now researchers from the UCSF School of Pharmacy and Stanford University have developed a device allowing them to study biofilms as they are exposed to concentrations of antibiotics much as would occur in the human body with normal dosing. The simulator includes specialized microscopy that allows the scientists to view dosing effects over time and in 3D—on different biofilm layers—down to the level of individual cells.
This new laboratory (in vitro) simulator to study antibiotics’ effects on biofilms is described and demonstrated in a recent paper in Antimicrobial Agents and Chemotherapy that was senior-authored by Katherine Yang, PharmD, MPH, a faculty member in the School’s Department of Clinical Pharmacy. The technique provides an important first step toward more effective biofilm treatments.
Journal citation: Haagensen JAJ, Verotta D, Huang L, Spormann A, Yang K, “New in vitro model to study the effect of human simulated antibiotic concentrations on bacterial biofilms,” Antimicrobial Agents and Chemotherapy, July 2015, Vol. 59, p. 4074-4081.
The challenge
Bacterial biofilms can form on inserted medical devices, such as catheters, breathing tubes, or orthopedic implants (e.g., knee/hip replacements), and on bodily tissues, such as heart valves (where they may cause infectious endocarditis).
Indeed, Pseudomonas aeruginosa, a bacteria residing primarily in biofilms, is the leading cause of illness and death in patients with cystic fibrosis (CF). The genetic disorder affects about 30,000 Americans and results in thick mucus in the airways on which biofilms can establish chronic, resistant infections with acute flare-ups that increasingly damage the lungs.
Biofilms thwart antibiotics by multiple means that can include:
- Enclosing themselves in a secreted slime that limits drug penetration.
- Cells in inner layers entering a non-growth state in response to limited oxygen and nutrients, which limits the efficacy of some commonly used antibiotics that work by inhibiting bacterial replication.
- Gaining or expressing antibiotic-resistant genes, due to sublethal exposure of bacteria to antibiotics within the aggregations or because of changes in gene activity when the bacteria live in communities.
To optimize antibiotic dosing, researchers seek to measure the drugs’ effect on biofilm killing. But this poses several challenges:
- Standard measures of antibiotic efficacy, such as estimating surviving cells per milliliter in liquid cultures, cannot be done in biofilms without disrupting their structures. (In the case of P. aeruginosa, biofilm communities appear as mushroom-like structures with a “cap” attached to a surface with a “stalk.”)
- A dynamic simulator to test the effect of antibiotics against biofilms (pharmacodynamics) must also mimic the changing concentration of a drug as it is absorbed and eliminated by the body (pharmacokinetics).
- Since biofilms are sensitive to factors such as temperature, nutrients, oxygen, and the fluid flow rate, those must remain constant in order to correctly distinguish the effects of the drug from changes in the environment.
The Research
Yang and her colleagues demonstrated a “gold standard” simulator that combines flow-cell technology with confocal laser-scanning microscopy, which allows them to directly see the effects of antibiotic dosing on different subpopulations of bacteria within each layer of the biofilm over the course of the dosing interval, thus showing 3D temporal effects of a drug.
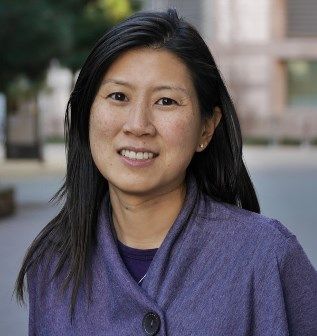
Katherine Yang, PharmD, MPH
For their device demonstration, the scientists simulated the effects of a single dose (bolus) of the antibiotic meropenem injected intravenously against biofilms of P. aeruginosa, including those grown from a standard laboratory strain of the bacteria and from bacteria taken from the infected lungs of a cystic fibrosis patient. (Meropenem is often used to treat multi-drug resistant P. aeruginosa, especially in CF patients.)
The simulation took into account the amount of drug that would not be bound with blood proteins (free drug concentration) as well as declining concentrations over an eight-hour period, which were based on the meropenem elimination rates found in studies of healthy volunteers.
In addition to examining drug effects on biofilms of P. aeruginosa, which is classified as a serious threat to human health by the U.S. Centers for Disease Control and Prevention, the preliminary experiments compared the effects of the dose over time on younger (thinner) biofilms compared to older (thicker) ones, which was recently cited as an urgently needed area of research by the European Society for Clinical Microbiology and Infectious Diseases.
The results
The study’s demonstration found the lowest concentration of antibiotics needed to inhibit notable overnight growth of P. aeruginosa biofilms was four to sixteen times greater than the equivalent needed to do the same for free-swimming bacteria. The experiments also showed different responses to antibiotics within different subpopulations of bacteria in the biofilm.
To further explore these results, it’s important to distinguish between antibiotic resistance and antibiotic tolerance. While antibiotic resistance reflects genetic changes that allow bacteria to continue to grow in the presence of antibiotics (e.g., some bacteria express enzymes that deactivate beta-lactam antibiotics, such as penicillin), antibiotic tolerance as seen in biofilms renders drugs less effective by other means, such as dormant subpopulations of bacteria, especially among the deeper layers.

Sequential images show effect of human-simulated concentrations of a two-gram intravenous bolus of meropenem on 72-hour-old microfilms of P. aeruginosa grown in simulator flow cells at times of drug exposure (left to right): zero (before drug), half-hour, one hour, four hours, and eight hours.
Green glow indicates living bacteria, while red staining marks dead cells. Top row shows live and dead cells, bottom row shows dead cells only. Main/upper left images look down at the biofilms (note caps of mushroom-like structures), the lower/right-hand images show cross-sections. At eight hours (far upper right), lower layers of biofilms remain green and thus viable.
The researchers’ microscopy confirmed increased antibiotic tolerance by older (thicker) biofilms grown for 72 hours (with cells in inner layers surviving the meropenem dose, unlike those at the periphery), as opposed to younger (thinner) biofilms grown for 24 hours, in which all layers were killed off within four hours of drug exposure. But the scientists found that when individual cells taken from the biofilms were grown in liquid cultures, their antibiotic susceptibility was unchanged. This indicates that the cells had not developed antibiotic resistance, but had increased their tolerance to antibiotics when living as a biofilm.
Yang and her co-authors write that such in vitro simulations are important first steps toward establishing optimal dosing regimens against biofilms. They note that future studies would include additional classes of antibiotics targeting P. aeruginosa, as well as combination drug therapies targeting different regions of the biofilm.
The new simulator apparatus could also be used to study other clinically significant biofilms, such as Staphylococcus aureus, as well as the effect of antibiotics on mixed bacterial communities and with other drug pharmacokinetic profiles and dosing strategies.
Tags
Topics:
Category:
Sites:
School of Pharmacy, Department of Clinical Pharmacy, PharmD Degree Program
About the School: The UCSF School of Pharmacy aims to solve the most pressing health care problems and strives to ensure that each patient receives the safest, most effective treatments. Our discoveries seed the development of novel therapies, and our researchers consistently lead the nation in NIH funding. The School’s doctor of pharmacy (PharmD) degree program, with its unique emphasis on scientific thinking, prepares students to be critical thinkers and leaders in their field.