- About
- Organization
- Organization Overview
- Dean’s Office
- Department of Bioengineering and Therapeutic Sciences
- Department of Clinical Pharmacy
- Department of Pharmaceutical Chemistry
- Quantitative Biosciences Institute
- Org Chart
- Research
- Education
- Patient Care
- People
- News
- Events
Fragment-based discovery: using smaller molecules to solve larger challenges
By David Jacobson / Thu Mar 24, 2016
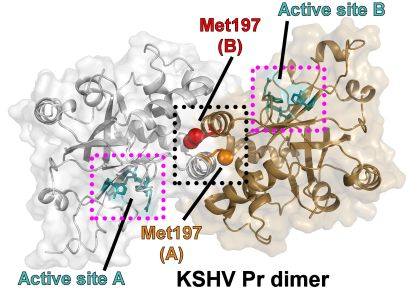
Protease enzymes of the Kaposi’s sarcoma-associated herpesvirus (KSHV Pr) require two monomer proteins to combine into a dimer in order to activate. Dotted black box is the protein-protein interface targeted for disruption by Craik Lab researchers. (Colored balls represent amino acids—methionines—labeled to help determine if screened fragments inhibit dimerization.)
To discover new drugs and chemical probes, researchers have traditionally screened small molecules—small enough by weight to pass through cell membranes. Their goal is typically to find compounds that selectively bind to a much larger protein molecule (often an enzyme) at a chemically reactive pocket known as the active site, inhibiting its activity to treat a disease or to better understand a biological process.
But scientists are increasingly testing libraries of so-called fragments—less complex molecules that are about half the weight of small molecules. These fragments alone won’t bind strongly enough and are typically not large enough to fully occupy an active site and serve as inhibitory drugs or probes, but they can provide key starting points for further development.
Recently, two studies by researchers in the School’s Department of Pharmaceutical Chemistry have used such smaller-than-small molecules to tackle big challenges:
- Discovering molecular probes to selectively inhibit a specific sub-set of enzymes that regulate gene expression—and go awry in cancers—while also pioneering the use of computational fragment docking to design such probes.
- Inhibiting a difficult-to-drug enzyme in a family of debilitating viruses, while also creating a case study in tackling protein-protein interactions.
Journal citation: Gable JE, Lee GM, Acker TM, Hulce KR, Gonzalez ER, Schweigler P, Melkko S, Farady CJ, Craik CS, “Fragment-based Protein-Protein Interaction Antagonists of a Viral Dimeric Protease,” ChemMedChem, Jan. 28, 2016, doi:10.1002/cmdc.201500526
Journal citation: Korczynska M, Le DD, Younger N, Gregori-Puigjane E, Tumber A, Krojer T, Velupillai S, Gileadi C, Nowak RP, Iwasa E, Pollock SB, Ortiz Torres I, Oppermann U, Shoichet BK, Fujimori DG, “Docking and Linking of Fragments to Discover Jumonji Histone Demethylase Inhibitors,” Journal of Medicinal Chemistry, Feb. 25, 2016, doi: 10.1021/acs.jmedchem.5b01527
Fragment-based discovery of viral protein interface inhibitors
An ongoing focus in the lab of Charles S. Craik, PhD, has been developing treatments for the herpesviruses that infect 90 percent of the world’s population. Current therapies can have limited effectiveness and face both rising rates of viral resistance and dose-limiting toxicity.
In otherwise healthy people, the nine human herpesviruses cause diseases such as chickenpox and shingles, mononucleosis, and oral and genital herpes. But in infants or adults with compromised immune systems, the infections can be devastating. For example, Kaposi’s sarcoma-associated herpes virus (KSHV) causes the most common cancer in patients with AIDS. Cytomegalovirus can cause life-threatening pneumonia and potentially disabling inflammatory diseases of the eyes, ears, and brain.
To develop new treatments for these viruses, the Craik Lab has been targeting their protease enzymes. As in all living things, these viral proteases play a key biological role by cleaving other proteins—in this case, providing materials the virus needs to replicate itself.
The shallow active sites of herpesvirus proteases have proven difficult to bind and inhibit. But using KSHV proteases as a model, Craik Lab researchers have found that these viral enzymes have an Achilles’ heel that could make them ripe for drug targeting: They only activate when a pair (separately called monomers) come together to form a dimer.
In fact, Craik Lab scientists have shown that binding a site at the interface of the two monomer proteins prevents them from undergoing changes in shape necessary for them to combine and activate. And since the proteases in all human herpesviruses are similar in their structure and mode of activation, such a dimer disrupter might be the basis for treating all nine pathogens.
As described in a paper published in a special January 2016 issue of the journal ChemMedChem devoted to protein-protein interactions, Craik et al sought to efficiently search for chemical starting points (scaffolds) for synthesizing molecules to disrupt KSHV protease dimerization. They began by screening more than 16,000 fragments for any ability to inhibit KSHV protease activity. Further screening selected the most potent of the inhibitors and then NMR spectroscopy was used to map precisely where these fragments were binding to the viral enzymes and thus how they inhibited their activity.
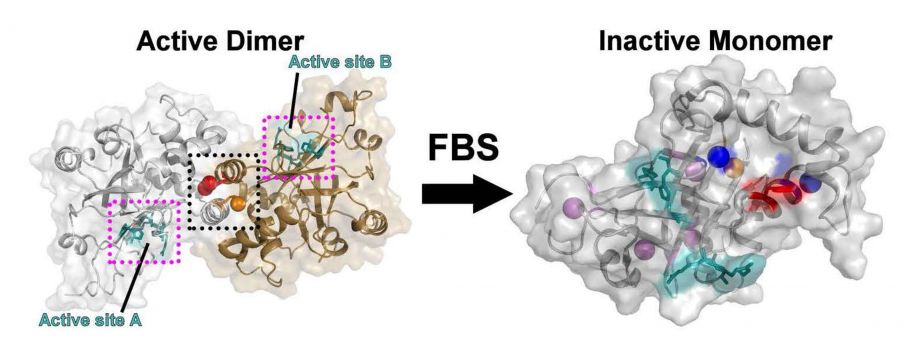
Fragment-based screening (FBS) was used to find compounds that inhibit protein-protein interactions that yield an activated human herpesvirus protease with a combined dimer structure. Red spot on inactive monomer at right indicates site targeted by researchers to prevent monomers from combining.
Since more than 80 percent of proteins are predicted to function in complexes, the herpesvirus proteases offer a case study for an even larger purpose—using a fragment screening approach to modulate protein-protein interactions in order to better understand biology and treat diseases.
Notably, the researchers found:
- Two classes of fragment compounds were relatively potent inhibitors of KSHV protease dimerization. Those and others offered promising scaffolds for further inhibitor development.
- The most potent inhibitors also reduced activity by cytomegalovirus proteases suggesting the potential to target other human herpes proteases.
- Among the 16,000 fragments screened, none blocked the KSHV protease active sites, further supporting the Craik Lab approach of targeting an alternative (allosteric) site at the interface of the twinned enzymes.
Fragment-based docking to develop probes of a cancer-related enzyme
Research in the lab of Danica Fujimori, PhD, focuses on the mechanisms and biological effects of enzymes that add or remove methyl groups (a carbon atom linked to three hydrogen atoms) from other protein molecules in cells. This modification alters how those proteins behave and, when it goes awry, is associated with a wide range of diseases—especially certain cancers—making the modifying enzymes prime drug therapy targets.
In particular, Fujimori Lab researchers study one subset of those enzymes that remove methyl groups (demethylases) from a specific amino acid (lysine; symbol K) on histones—proteins that package each cell’s DNA, which wraps around them like thread on spools. Such histone modification can result in genes being turned off and on. (The study of this genomic regulation is called epigenetics.)
More than two dozen members of a histone lysine demethylase (KDMs) family of enzymes have been discovered in humans in the past decade. Increased activity by members of a subfamily dubbed KDM4 is thought to promote tumor formation, especially in prostate cancer, but also in colon cancer and some types of breast cancers.
To better understand the role of KDM4 enzymes in normal biology and in cancers, Fujimori’s lab seeks to develop new chemical probes, small molecules that bind to their catalytic sites (ligands) both strongly and selectively (e.g., not to related KDM2 or KDM6) and inhibit their activity.
Enter Brian Shoichet, PhD, whose lab’s research includes the ongoing improvement of docking computer programs that virtually screen potential ligands for the strength (affinity) with which they bind to the 3-D structure of a target protein’s active site in a given orientation. Department researchers, including Shoichet, led by now-emeritus faculty member Irwin “Tack” Kuntz, PhD, developed the first such program in the 1980s. Dubbed UCSF DOCK, it has been improved via 14 versions since. Nowadays, such computational screening is commonly used in drug discovery worldwide.
Shoichet and department colleague John Irwin, PhD, also developed and maintain a database called ZINC containing the 3D structures of 90 million compounds, including more than 600,000 fragments, which are formatted for docking and also commercially available for follow-up non-virtual testing.
In a paper published in a special February 2016 issue of the Journal of Medicinal Chemistry devoted to “Novel Therapeutics Targeting Epigenetics,” the researchers docked the ZINC library of fragments into the structure of KDM4A enzymes experimentally determined by the study’s co-authors at the University of Oxford.
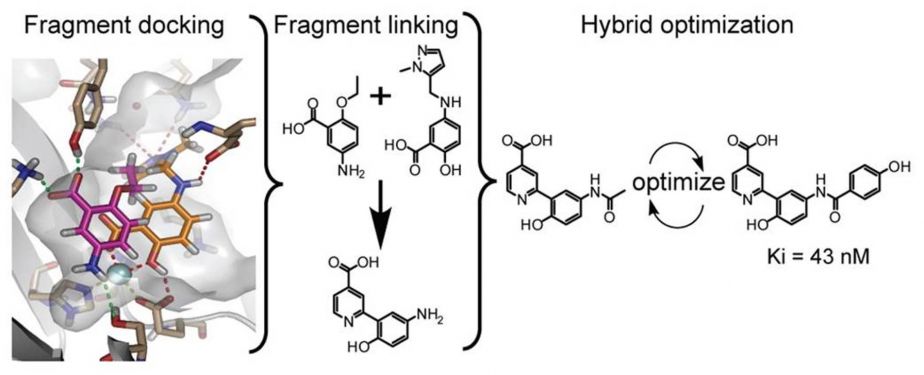
(Left to right) Fragment docking: Computer-based docking of fragments in KDM4 demethylase active sites discovered binding fragments; Fragment linking: these were fused to create small molecules; Hybrid optimization: molecules were chemically optimized to maximize selective inhibition.
On average, each fragment was docked in nearly 22,000 orientations and with 42 conformations (molecules shift in shape), yielding about 1.5 trillion computer-calculated combinations of fragments with KDM4A’s active site. The docked fragments were scored and ranked for their binding strength. Fourteen such prioritized fragments strongly bound and inhibited KDM4, indicating a successful use of fragment docking for enzymes with few previously known ligands.
And in a first, the researchers used the docking results—specifically, the orientations of the strongly binding fragments—to guide the subsequent design of small molecules. They fused the fragments to create hybrid compounds with up to 700 times greater inhibitory effect than their parent fragments.
Further improvements in binding affinity were achieved through ensuing chemical synthesis, experimentation, and optimization. The newly created ligands, while not perfectly selective for the KDM4 subfamily alone, do not inhibit oxygen-sensing enzymes related to demethylases (a weakness of prior probes), nor several other subfamilies of demethylases. Thus, they provide the basis for further development of these vital molecular tools.
Tags
Topics:
Category:
Sites:
School of Pharmacy, Department of Pharmaceutical Chemistry, PharmD Degree Program
About the School: The UCSF School of Pharmacy aims to solve the most pressing health care problems and strives to ensure that each patient receives the safest, most effective treatments. Our discoveries seed the development of novel therapies, and our researchers consistently lead the nation in NIH funding. The School’s doctor of pharmacy (PharmD) degree program, with its unique emphasis on scientific thinking, prepares students to be critical thinkers and leaders in their field.