- About
- Organization
- Organization Overview
- Dean’s Office
- Department of Bioengineering and Therapeutic Sciences
- Department of Clinical Pharmacy
- Department of Pharmaceutical Chemistry
- Quantitative Biosciences Institute
- Org Chart
- Research
- Education
- Patient Care
- People
- News
- Events
Jamming the gears of a deadly virus
Wells and Kortemme lead two efforts to develop therapies against SARS-CoV-2
By Levi Gadye / Fri Nov 13, 2020
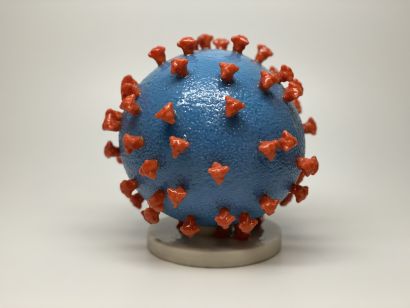
NIH
A 3D printed model of a SARS-CoV-2 viral particle (blue) dotted with the spike protein (red) that enables the virus to infect human cells. The spike protein is the target of research efforts to prevent and treat COVID-19.
When San Francisco’s shelter-in-place order was announced on March 16 to combat the spread of COVID-19, UC San Francisco postdoctoral scholars Anum Glasgow, PhD, and Jeff Glasgow, PhD, found themselves confined to their apartment, temporarily barred from their UCSF laboratories. But the married couple put their isolation to good use.
SARS-CoV-2, the virus that causes COVID-19, uses “spike proteins” to home in on receptors on human cells, leading to infection. Researchers at the Chinese Academy of Sciences had just published the shapes, or structures, of the spike protein stuck to the human receptor. For the Glasgows, who are both protein engineers, “it was hard not to be thinking about these structures all the time in the middle of a pandemic,” said Anum Glasgow.
The Glasgows weren’t alone. James Wells, PhD, faculty member in the School’s Department of Pharmaceutical Chemistry, an expert in protein therapeutics, knew that his lab, and many others at UCSF, were perfectly suited to designing drugs that could stop SARS-CoV-2 at the level of its molecular spike. He would soon be overseeing a variety of efforts toward this goal.
“Viruses like these are dynamic, so having multiple ways of defeating them is useful,” said Wells.
Anum Glasgow dreamed of turning the barb into the virus’s Achilles’ heel. She works in the laboratory of Tanja Kortemme, PhD, faculty member in the Department of Bioengineering and Therapeutic Sciences (BTS), a joint department of the UCSF Schools of Pharmacy and Medicine.
The Kortemme Lab quickly joined forces with the Wells Lab, and within weeks, the Glasgows, along with dozens of colleagues across the Wells, Kortemme, and other UCSF labs, were diligently designing and testing molecules to block the spike.
In late October, the fruits of these efforts—two unique approaches to stopping SARS-CoV-2 by gumming up its spike—were published in the Proceedings of the National Academy of Sciences (PNAS) and Nature Chemical Biology, respectively.
“This was such a team effort across many labs,” said Wells, senior author on both papers. “It really shows what’s possible when you have the right people and the right motivation to solve a big problem.”
Neutralizing SARS-CoV-2’s devious spike
SARS-CoV-2 uses its distinctive spike to latch onto a receptor called ACE2 on the surface of a human cell. Once there it prompts the human cell to ferry the virus inside. Then, the virus co-opts human enzymes to make copies of itself and spread to other cells.
Another UCSF scientist who was inspired to interfere with the viral spike was Shion Lim, PhD, who works at Genentech but was a postdoc in the Wells Lab at the time. Lim teamed up with Wells Lab graduate student Cole Bracken, who had just finished building a library of ten billion small antibody fragments, known as VH domains, that could be screened for their ability to bind to any specific protein.
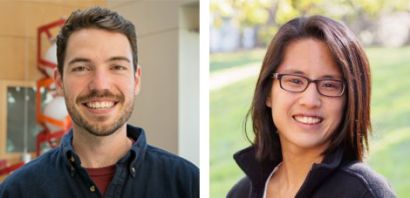
Colton Bracken, left, and Shion Lim, PhD, led the VH domain project.
“Within a week of lockdown, we were discussing what we could do, since we had everything ready to go,” said Lim. “Jim [Wells] has experience with therapeutics, we have active collaborations with industry, and we knew we could make a real impact.”
“It’s kind of like a fishing experiment,” said Bracken. “We threw the spike protein into this proverbial fishing pond of VH domains and pulled out things that stuck to it,” wagering that those molecules might disable the virus.
Meanwhile, the Glasgows were working on their own spike jammer, pondering how to stop the virus using decoy versions of the human ACE2 receptor. Anum Glasgow explored 3D renderings of the spike protein and ACE2 receptor on her computer, tweaking the receptor to make it bind to the virus even more strongly than the normal ACE2 receptor. This sort of strategy is known as a receptor trap, because it captures pathogens away from human cells.
“We were stuck in the house, wishing we could do science, and Anum was able to get some interesting data on the computer to start things out in a week or two,” said Jeff Glasgow, who works in the Wells Lab. “And then we were able to go into the lab and actually make the molecules.”
As people nationwide learned to bake sourdough and adapted to remote work in late March, the Wells and Kortemme Labs had reopened for the important business of discovering new therapies for COVID-19. But the process of doing science had changed, and making discoveries during a pandemic would now take a whole new level of collaboration and teamwork.
A village for science
Finding molecules that could grab hold of the spike protein and disable SARS-CoV-2 requires a multidisciplinary team. Even between Kortemme’s expertise with the computational design of proteins and Wells’ expertise with protein engineering and testing, the group of scientists would need outside help—particularly when it came to actually working with a dangerous virus.
The Glasgows’ project, a joint effort of the Wells and Kortemme labs, would go from virtually designing a few varieties of ACE2 receptor trap, to improving on those molecules with a process called directed evolution in petri dishes, and then validating their ability to stick strongly to the spike protein.
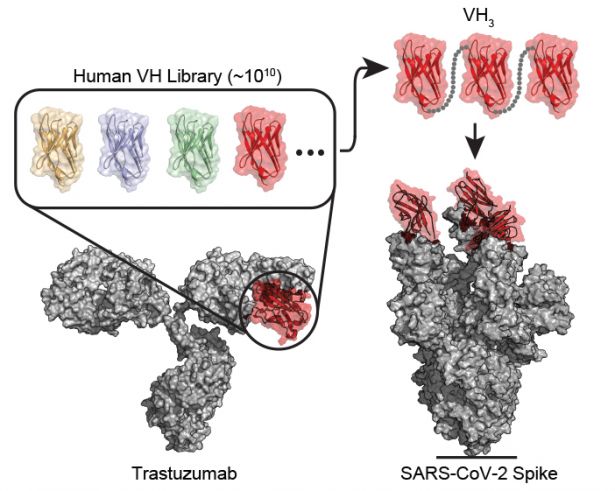
Colton Bracken/Wells Lab
School scientists developed a potential COVID-19 therapy out of an antibody fragment, called the VH domain, that disables the spike protein of SARS-CoV-2. Left: a depiction of the VH domain library, containing billions of variants of a small portion of the antibody drug trastuzumab. Right: three copies of the stickiest VH domain were linked together to more effectively block the spike.
Meanwhile, Bracken and Lim’s VH domain project, a project of the Wells Lab, followed a comparable path: fish out any antibody fragments that stuck to the spike protein, tweak them to improve their stickiness, and confirm that they do interfere with the spike’s binding to the ACE2 receptor. The team found that linking three copies of the stickiest antibody fragment made for an even better blocker of the spike protein.
The best receptor traps and antibody fragments would be tested first against benign pseudoviruses—a sort of “model of the virus that isn’t as dangerous,” according to Wells—and ultimately, active SARS-CoV-2.
These final experiments with active SARS-CoV-2 would need to be carried out in a secure facility designed for experiments with dangerous, infectious agents, something neither the Kortemme nor Wells Lab could execute themselves.
Both projects made it across the finish line with such high-stakes experiments thanks to collaborators in the UCSF Quantitative Bioscience Institute’s Coronavirus Research Group (QCRG). Kliment Verba, PhD, and Oren Rosenberg, MD, PhD, assisted with the design of these molecular therapies as members of the leadership team of the QCRG Structural Biology Group. Rosenberg, who is a faculty member in the School of Medicine, also facilitated experiments with the VH domain and the live virus. The Quantitative Biosciences Institute (QBI) is an Organized Research Unit in the School of Pharmacy.
The laboratory of Tom Hobman, PhD, at the University of Alberta, performed live virus experiments for the ACE2 receptor trap project.
“It was a terrific collaboration between people who were experts in all of these different methods,” said Kortemme. “And as soon as the problem was defined, everybody went to work immediately and applied these foundational methods, developed over decades, to carry out this work incredibly quickly.”
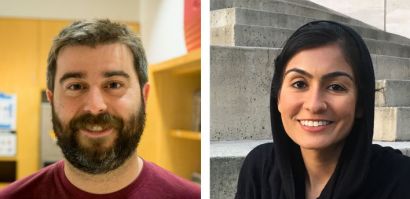
Jeff Glasgow, PhD, left, and Anum Glasgow, PhD, led the ACE2 receptor trap project.
New collaborations happened within individual labs, too. With workplace capacity initially limited to 12.5 percent of normal to prevent the spread of COVID-19, the Glasgows, Lim, Bracken, and all their colleagues had to change how they worked. Scientists, whether they are graduate students, technicians, or postdocs, normally juggle a variety of experiments of their own. Now, limited to coming into the lab only a couple days per week, they had to pitch in on each other’s work.
“We would have four to five people come into the lab per day, and those people would do a bunch of little tasks for everyone, to keep the experiments of dozens of people moving forward,” said Jeff Glasgow. “So we definitely were working fairly long hours.”
Lim juggled work on both the ACE2 receptor trap and the VH domain projects. And these two projects weren’t the only ones occurring in either lab, they were just the first two therapeutic projects to succeed.
“There were other things we tried to block the spike protein that didn’t work,” said Bracken. “It was a great effort by Jim and the postdocs, like Shion, who were looking down at all these projects, making sure we worked on the most promising leads for new therapies.”
Microscopic solutions for a global problem
By May, the two teams had optimized their respective ACE2 receptor trap and VH domain blockers of SARS-CoV-2 and tested them against authentic, active SARS-CoV-2.
Both strategies worked in the lab, obstructing the spike protein and preventing the virus from binding to the ACE2 receptor and getting into human cells. The turnaround, from designing the experiments to proving the effectiveness of these potential therapies and submitting the results, was just four months.
“This was lightspeed engineering, and all of us should be super proud of that,” said Wells. “There’s no doubt that we can respond quickly, especially when the need is so great.”
Wells is now shepherding both projects along the path to clinical trials. There are many contenders for the next therapy for COVID-19, developed both by pharmaceutical companies and academic labs, according to Wells, but that’s a good thing.
“The pandemic could go on for years,” he said. “We need to have an armamentarium of binders [to the spike protein] to really get this under control.”
For the graduate students and postdocs behind these potential therapies, the success has given them a new perspective on the real-world value of discovery sciences—science borne first out of curiosity that serves as the foundation for therapies and technologies that can change the world.
“Jim likes to call our lab a little pirate ship, and then he calls these big pharmaceutical companies big old battleships,” said Bracken. “We just want to try to make an impact however we can, with the awesome talent and small size of our lab.”
Additional authors on the VH domain study were Paige Solomon; Nicholas J. Rettko; Duy P. Nguyen, PhD; Beth Shoshana Zha, MD, PhD; Kaitlin Schaefer, PhD; James R. Byrnes, PhD; Jie Zhou, PhD; Irene Lui; Jia Liu, MSc; Katarina Pance; QCRG Structural Biology Consortium; Xin X. Zhou, PhD; and Kevin K. Leung, PhD.
Additional authors on the ACE2 receptor trap study were Daniel Limonta, PhD; Paige Solomon; Irene Lui; Yang Zhang, PhD; Matthew A. Nix, PhD; Nicholas J. Rettko; Shoshana Zha, MD, PhD; Rachel Yamin, PhD; Kevin Kao; Oren S. Rosenberg, MD, PhD; Jeffrey V. Ravetch, MD, PhD; Arun P. Wiita, MD, PhD; Kevin K. Leung, PhD; and Xin X. Zhou, PhD.
More
Bi-paratopic and multivalent VH domains block ACE2 binding and neutralize SARS-CoV-2 (Nature Chemical Biology)
Engineered ACE2 receptor traps potently neutralize SARS-CoV-2 (PNAS)
Tags
Topics:
Category:
Sites:
School of Pharmacy, Department of Pharmaceutical Chemistry, Department of Bioengineering and Therapeutic Sciences, PharmD Degree Program
About the School: The UCSF School of Pharmacy aims to solve the most pressing health care problems and strives to ensure that each patient receives the safest, most effective treatments. Our discoveries seed the development of novel therapies, and our researchers consistently lead the nation in NIH funding. The School’s doctor of pharmacy (PharmD) degree program, with its unique emphasis on scientific thinking, prepares students to be critical thinkers and leaders in their field.